Add Your Heading Text Here
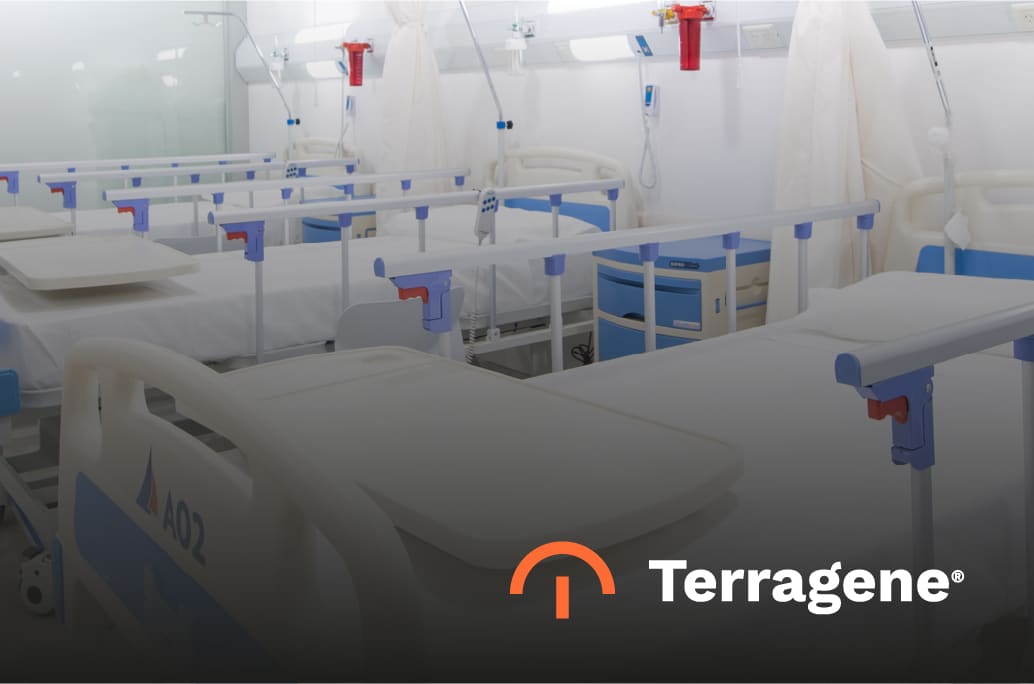
Room Disinfection: which technology should I choose?
By Dr. Nicolas Hedin
In 1991 a study by Weinstein revealed that healthcare-associated infections – or HAI were 40 to 60% caused by patient’s endogenous flora, between 20 to 40% through the hands of hospital personnel and approximately a 20% of the cases were due to environmental, food or air contamination (1). There is mounting evidence pointing towards the importance of ambient cleaning/disinfection to prevent and control HAI in hospitals (Dancer, 2009). The common HAI-causing pathogens have the innate ability to survive on different surfaces inside the hospital for long periods of time (Dancer 1999) (Figure 1). Among these organisms, the most common are: Clostridium difficile, meticillin-resistant Staphylococcus aureus (MRSA), vancomycin-resistant enterococci (VRE), Acinetobacter spp. and norovirus (2).
Scientific evidence shows that contaminated surfaces contribute positively to the endemic transmission of most of these pathogens. Admission of a healthy patient to a room previously occupied by a VRE, MRSA or Clostridium difficile infected person, increases the chances of HAI infectionby at least a factor of two (3). There is also a study showing that improving room disinfection reduces the increased risk of HAI infection (4). Zimlichman et al. estimated in the year 2013 that 9.8 billion dollars per year are spent due to HAI infections. It is thus clear that proper environmental disinfection is a key point when discussing healthcare (5).
In the year 2011 Manian and collaborators (6) demonstrated that rooms contaminated with multidrug-resistant Acinetobacter baumannii complex and MRSA were difficult to clean and even after four rounds of cleaning/disinfection with bleach, the pathogens remained in the room. The authors concluded that the suboptimal conditions of the cleaning/disinfection process per se are the causes of these results rather than the ineffectiveness of the disinfectant. Several other studies point toward the same direction (7 – 10) and highlight the importance of the human factor. Finding the proper protocol seems mandatory, and educating the personal is also a key point, as this might help them understand the importance of their activity. It is also a fact that modifying human behavior is sometimes a difficult task: while the education process is active and on-going, the results are positive but after some months they return to the starting point (11 – 12). In this sense, the development of the “no-touch automated room disinfection” (NTD) technology helps achieving proper cleaning and disinfection standards. But …how many flavors do we have?
In the last years several NTD systems have been developed and the most tested and useful ones rely on hydrogen peroxide H2O2 or ultra-violet radiation (UV). Both technologies are nowadays in the spotlight, as they have been proposed as accepted methods for N95 masks decontamination, according to CDC (https://www.cdc.gov/) given the actual context of COVID-19 where there is a shortage of N95 masks. It seems clear that H2O2 microbicidal activity is due to the oxidation of pathogen’s molecules and also through the formation of free radicals and other reactive species, although further studies are required (13). UV mechanisms are well studied, and the microbicidal effect is given by the un-stabilization of certain purines, pyrimidines and flavin bonds, thus affecting DNA and RNA leading to their dimerization (14). NTD technology has grown in the last years and several different options were developed, in the next paragraphs I will discuss the most popular approaches used nowadays: aHP, H2O2 vapour, UVC and mixed O3/H2O2 technologies.
aHP stands for aerosolized hydrogen peroxide. This technology delivers H2O2 generated by pressure from a solution with a relatively low concentration of the active principle (5-6% H2O2). Peroxide is delivered together with approximately 50 ppm of silver cations which are thought to help stabilizing hydrogen peroxide and also improving bactericidal efficacy by binding with disulfide (S-S) and sulfhydryl (-SH) groups found in proteins from the bacterial cell-wall (15 – 17). The Ag+2 ions keep the O-2 ions. Different particle sizes can be generated according to the setup of the equipment manufacturer (from 0.5 to 10 µm) (18, 19) generating the called ‘dry-mist hydrogen peroxide’, which is naturally decomposed to oxygen and water after exposure. There are several studies stating that 4-log reduction of C. difficile is possible, but no satisfactory results (with standard equipment) are obtained when 6-log spore biological indicators are used (20). Recently, an aHP based system claimed a 6-log reduction of Geobacillus stearothermophilus by means of 12% plasma-activated H2O2 (21). In general terms aHP is an easy-to-use technology and it is the cheapest one, but depending on the room size, multiple aHP units may be required. Sealing doors and air vents is mandatory and the process extends from 2 to 4 hours depending on the amount of cycles required.
Vaporized H2O2 (vH2O2) is the second and one of the most proven options. This technology uses heat (130°C) to vaporize a 30 – 35% hydrogen peroxide solution. vH2O2 achieves higher amounts of active H2O2 (150 – 750 ppm) compared to aHP (less than 160 ppm) and generates homogeneous vapors throughout the room to be decontaminated. Two different approaches can be mentioned: HPV for hydrogen peroxide vapor and VHP for vaporized hydrogen peroxide. HPV generates a saturated peroxide hydrogen atmosphere that condensates on surfaces (22, 23), while VHP does not generate condensation. Both systems were repeatedly proven to be effective against hard to kill bacteria (C. difficile spores, MRSA, VRE, A. baumannii and norovirus) and Geobacillus stearothermophilus BI spores. This is the chosen option when there are pathogens’ outbreaks (i.e. C. difficile and A. baumannii) (24). HPV technology has the disadvantage of using two separate units, the generator unit and the aeration unit, which makes it more complex. In general, vaporized technology needs a minimum of training of the personnel who will be in charge of the following tasks: seal the room to be disinfected in order to avoid H2O2 leakage; control the equipment and measure remaining peroxide on the room prior to re-entrance (by means of a hand-held monitor). Cycles vary in time, as they depend on the room-size, but they can last between 2 to 8 hours.
UV radiation can be divided into three groups according to its wavelength: UVA from 315 – 400 nm, UVB 280 – 315 nm and UVC from 200 – 280 nm. UVC is absorbed by nucleic acid and thus is the most lethal option for microorganisms (25). UVC radiation needs to be in direct contact with the surface to be disinfected, and thus the system is most often capable to automatically “move” around the room to be disinfected. In this context, UVC has been proposed as a reliable method to decontaminate N95 filtering face-piece respirators (FFRs) (26). 3 – 5 log reduction of H1N1 influenza virus was achieved using UVC technology (26, 27). Apart from regular UVC continuous light systems, there is also another technology based on Xenon lamps, which uses light pulses and is called PX-UV. There is no consensus about which technology is better as there are relatively few reports about pulsed technology (28, 29). A main advantage of UV based technology compared to H2O2is the short time required for disinfection (10 – 45 minutes average) (30), and no sealing of the room is needed, saving even more time. On the other hand, UV disinfection process is more difficult to assess than peroxide based processes, as much of the disinfection effectiveness will depend on the distance to the light-emitting source and the nature of the incidence light (direct or indirect).
No-touch automated room disinfection technology is a solution for any place where HAI causing organisms might be present: Sterile Processing Departments (SPD or CSSD), healthcare facilities and industry also, but care should be taken to assess the correct functioning of these technologies as they are different one from the other (Table 1). Traditional microbiological approaches are not practical for routine analysis as they require sample cultivation and identification which is a time-consuming process and is expensive. There are alternatives to replace traditional test. On the one hand, commercially available biological indicators can be used, and as an example there are on the market fluorescence-based biological indicators which ensure the correct inactivation of a 1×106 spore population of Geobacillus stearothermophilus by means of hydrogen peroxide, giving a quick 1 hour readout so the room can be liberated almost instantly. There are also tridimensional chemical indicators that can be used to assure the correct exposition of the room to the peroxide hydrogen, both for aerosol and for vaporized hydrogen peroxide. As UV disinfection process is more difficult to assess, these processes should be carefully evaluated by employing indicators in every hard-to-reach place. There are chemical indicators and chemical dosimeters to ensure that a surface receives the correct UV-dose and a recently released 3D UV chemical indicator allows to assess the correct room UV-disinfection.
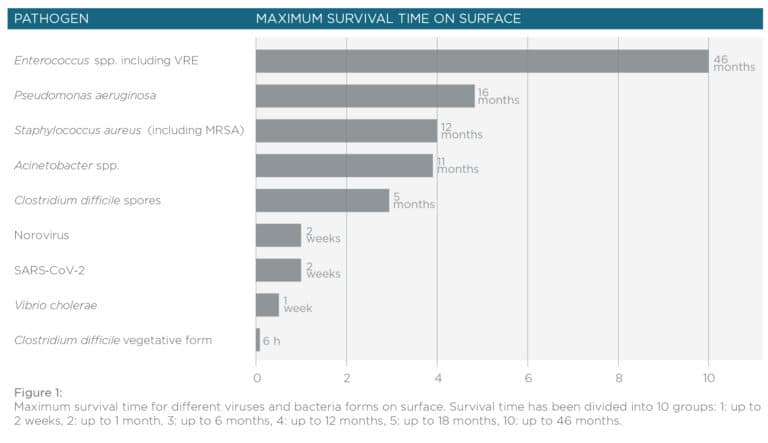
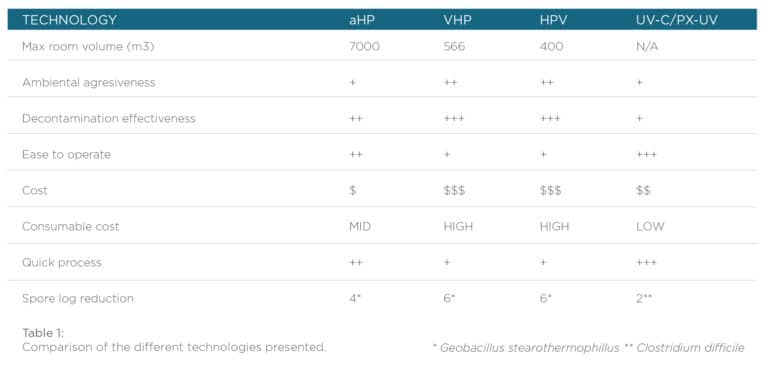
- Weinstein, R. A. (1991). Epidemiology and control of nosocomial infections in adult intensive care units. The American journal of medicine, 91(3), S179-S184.
- Dancer, S. J. (2009). The role of environmental cleaning in the control of hospital-acquired infection. Journal of hospital Infection, 73(4), 378-385.
- Otter, J. A., Yezli, S., & French, G. L. (2011). The role played by contaminated surfaces in the transmission of nosocomial pathogens. Infection Control & Hospital Epidemiology, 32(7), 687-699.
- Chemaly, R. F., Simmons, S., Dale Jr, C., Ghantoji, S. S., Rodriguez, M., Gubb, J., … & Stibich, M. (2014). The role of the healthcare environment in the spread of multidrug-resistant organisms: update on current best practices for containment. Therapeutic advances in infectious disease, 2(3-4), 79-90.
- Zimlichman, E., Henderson, D., Tamir, O., Franz, C., Song, P., Yamin, C. K., … & Bates, D. W. (2013). Health care–associated infections: a meta-analysis of costs and financial impact on the US health care system. JAMA internal medicine, 173(22), 2039-2046.
- Manian, F. A., Griesenauer, S., Senkel, D., Setzer, J. M., Doll, S. A., Perry, A. M., & Wiechens, M. (2011). Isolation of Acinetobacter baumannii complex and methicillin-resistant Staphylococcus aureus from hospital rooms following terminal cleaning and disinfection: can we do better?. Infection control and hospital epidemiology, 32(7), 667-672.
- Byers, K. E., Durbin, L. J., Simonton, B. M., Anglim, A. M., Adal, K. A., & Farr, B. M. (1998). Disinfection of Hospital Rooms Contaminated With Vancomycin-Resistant Entemcocms faecium. Infection Control & Hospital Epidemiology, 19(4), 261-264.
- French, G. L., Otter, J. A., Shannon, K. P., Adams, N. M. T., Watling, D., & Parks, M. J. (2004). Tackling contamination of the hospital environment by methicillin-resistant Staphylococcus aureus (MRSA): a comparison between conventional terminal cleaning and hydrogen peroxide vapour decontamination. Journal of Hospital Infection, 57(1), 31-37.
- Manian, F. A., Griesnauer, S., & Bryant, A. (2013). Implementation of hospital-wide enhanced terminal cleaning of targeted patient rooms and its impact on endemic Clostridium difficile infection rates. American journal of infection control, 41(6), 537-541.
- Wilcox, M. H., Fawley, W. N., Wigglesworth, N., Parnell, P., Verity, P., & Freeman, J. (2003). Comparison of the effect of detergent versus hypochlorite cleaning on environmental contamination and incidence of Clostridium difficile Journal of Hospital Infection, 54(2), 109-114.
- Fitzgerald, T., Sholtz, L. A., Marion, N., Turner, P., Carling, P. C., & Rupp, M. E. (2012). Maintenance of environmental services cleaning and disinfection in the ICU after a performance improvement project. American Journal of Infection Control, 40(5), e159.
- Rutala, W. A., & Weber, D. J. (2011). Are room decontamination units needed to prevent transmission of environmental pathogens?. Infection Control & Hospital Epidemiology, 32(8), 743-747.
- McDonnell, G. (2009). The use of hydrogen peroxide for disinfection and sterilization applications. PATAI’S Chemistry of Functional Groups, 1-34.
- Cutler, T. D., & Zimmerman, J. J. (2011). Ultraviolet irradiation and the mechanisms underlying its inactivation of infectious agents. Animal Health Research Reviews, 12(1), 15.
- Davoudi, M., Ehrampoush, M. H., Vakili, T., Absalan, A., & Ebrahimi, A. (2012). Antibacterial effects of hydrogen peroxide and silver composition on selected pathogenic enterobacteriaceae. International Journal of Environmental Health Engineering, 1(1), 23.
- Weber, D. J., Rutala, W. A., Sickbert-Bennett, E. E., Kanamori, H., Anderson, D., & CDC Prevention Epicenters Program. (2019). Continuous room decontamination technologies. American journal of infection control, 47, A72-A78.
- Freyssenet, C., & Karlen, S. (2019). Plasma-activated aerosolized hydrogen peroxide (aHP) in surface inactivation procedures. Applied Biosafety, 24(1), 10-19.
- Orlando, P., Cristina, M. L., Dallera, M., Ottria, G., Vitale, A., & Badolati, G. (2008). Surface disinfection: evaluation of the efficacy of a nebulization system spraying hydrogen peroxide. J Prev Med Hyg, 49(Suppl 3), 116-9.
- Barbut, F., Menuet, D., Verachten, M., & Girou, E. (2009). Comparison of the efficacy of a hydrogen peroxide dry-mist disinfection system and sodium hypochlorite solution for eradication of Clostridium difficile Infection Control & Hospital Epidemiology, 30(6), 507-514.
- Holmdahl, T., Lanbeck, P., Wullt, M., & Walder, M. H. (2011). A head-to-head comparison of hydrogen peroxide vapor and aerosol room decontamination systems. Infection Control & Hospital Epidemiology, 32(9), 831-836.
- Freyssenet, C., & Karlen, S. (2019). Plasma-activated aerosolized hydrogen peroxide (aHP) in surface inactivation procedures. Applied Biosafety, 24(1), 10-19.
- Hall, L., Otter, J. A., Chewins, J., & Wengenack, N. L. (2007). Use of hydrogen peroxide vapor for deactivation of Mycobacterium tuberculosis in a biological safety cabinet and a room. Journal of clinical microbiology, 45(3), 810-815.
- Ray, A., Perez, F., Beltramini, A. M., Jakubowycz, M., Dimick, P., Jacobs, M. R., … & Salata, R. A. (2010). Use of vaporized hydrogen peroxide decontamination during an outbreak of multidrug-resistant Acinetobacter baumannii infection at a long-term acute care hospital. Infection control and hospital epidemiology: the official journal of the Society of Hospital Epidemiologists of America, 31(12), 1236.
- Cooper, T., O’Leary, M., Yezli, S., & Otter, J. A. (2011). Impact of environmental decontamination using hydrogen peroxide vapour on the incidence of Clostridium difficile infection in one hospital Trust. Journal of Hospital Infection, 78(3), 238-240.
- Dai, T., Vrahas, M. S., Murray, C. K., & Hamblin, M. R. (2012). Ultraviolet C irradiation: an alternative antimicrobial approach to localized infections?. Expert review of anti-infective therapy, 10(2), 185-195.
- Mills, D., Harnish, D. A., Lawrence, C., Sandoval-Powers, M., & Heimbuch, B. K. (2018). Ultraviolet germicidal irradiation of influenza-contaminated N95 filtering facepiece respirators. American journal of infection control, 46(7), e49-e55.
- Heimbuch, B. K., Wallace, W. H., Kinney, K., Lumley, A. E., Wu, C. Y., Woo, M. H., & Wander, J. D. (2011). A pandemic influenza preparedness study: use of energetic methods to decontaminate filtering facepiece respirators contaminated with H1N1 aerosols and droplets. American journal of infection control, 39(1), e1-e9.
- El Haddad, L., Ghantoji, S. S., Stibich, M., Fleming, J. B., Segal, C., Ware, K. M., & Chemaly, R. F. (2017). Evaluation of a pulsed xenon ultraviolet disinfection system to decrease bacterial contamination in operating rooms. BMC infectious diseases, 17(1), 1-5.
- Nerandzic, M. M., Thota, P., Sankar, T., Jencson, A., Cadnum, J. L., Ray, A. J., … & Donskey, C. J. (2015). Evaluation of a pulsed xenon ultraviolet disinfection system for reduction of healthcare-associated pathogens in hospital rooms. infection control & hospital epidemiology, 36(2), 192-197.
- Weber, D. J., Rutala, W. A., Anderson, D. J., Chen, L. F., Sickbert-Bennett, E. E., & Boyce, J. M. (2016). Effectiveness of ultraviolet devices and hydrogen peroxide systems for terminal room decontamination: focus on clinical trials. American journal of infection control, 44(5), e77-e84.